- Home
- Publications
- PAGES Magazine
- Cosmogenic Nuclide Moraine Chronologies From Patagonia: A Globally Synchronic Response of Mountain Glaciers During Termination 1?
Cosmogenic nuclide moraine chronologies from Patagonia: A globally synchronic response of mountain glaciers during Termination 1?
Rodrigo L. Soteres, E.A. Sagredo, M.R. Kaplan, M.A. Martini, F.M. Riquelme and J.M. Schaefer
Past Global Changes Magazine
31(2)
108-109
2023
Rodrigo L. Soteres
1,2,
E.A. Sagredo3,4,
M.R. Kaplan5,
M.A. Martini6,
F.M. Riquelme3 and
J.M. Schaefer5,7
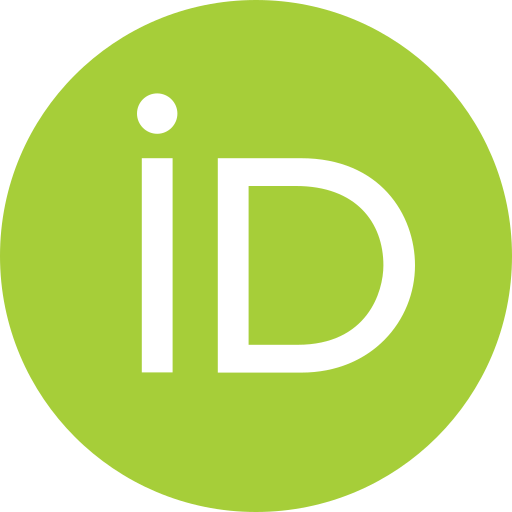
E.A. Sagredo3,4,
M.R. Kaplan5,
M.A. Martini6,
F.M. Riquelme3 and
J.M. Schaefer5,7
Surface-exposure dating of moraines reveals that Patagonian glaciers fluctuated at the pulsebeat mimicked in polar ice cores from both hemispheres. These findings favor hypotheses that invoke coupled oceanic–atmospheric drivers to generate and propagate millennial-scale climate shifts during Termination 1.
Moraines as past climate archives
Mountain glaciers are very sensitive to climate oscillations, especially to atmospheric temperature and precipitation. When climate conditions favor glacier growth, ice mobilizes massive quantities of sediments, including large erratic boulders, which are piled up alongside the ice margins, forming sharp and elongated hilly landforms, called moraines. We assume they form mostly when glaciers are in close equilibrium with the local prevailing climate, culminating at the transition between cold and warm periods. Therefore, well-preserved sequences of moraines are valuable archives of the timing of terrestrial paleoclimate.
Cosmogenic nuclides (or isotopes) are mostly generated by the interaction between cosmic radiation and target minerals within rock surfaces exposed to the atmosphere. Given that these nuclides are naturally near-absent in most lithologies, their concentration will start building up when the rock begins to intercept cosmic radiation products. So, if the production rate of these nuclides is known, we can measure them and then calculate the “exposure age” (Schaefer et al. 2022). Continual refinement of surface-exposure dating based on in situ terrestrial cosmogenic nuclides, particularly 10Be in moraine boulders, has allowed us to assign precise ages to former glacial oscillations and, therefore, to constrain the chronology of past climate variability.
The conceptual interpretation of 10Be glacier chronologies (Fig. 1a) assumes that boulders in resting position at the highest part of the moraine ridges represent the cessation of its construction, so the surface exposure age of these boulders will pinpoint the onset of glacier recession (Fig. 1b). We prefer to sample tall and large boulders well embedded in the top of the moraine to prevent potential post-depositional displacements and/or exhumation that could provide anomalously young ages. We also aim to collect samples from glacially polished surfaces to try reducing inherited cosmogenic nuclides generated during prior exposure episodes, which could yield anomalously old ages (Fig. 1c). This sample approach permits us to diminish geological uncertainties affecting surface-cosmogenic-nuclide concentrations and, thus, to obtain accurate ages for culminations of glacier pulses.
Patagonia: the southernmost mid-latitude continental landmass in the world
Patagonia, including Tierra del Fuego, spans between ~40° and ~56°S in South America, comprising both Chilean and Argentine territory (Fig. 2a). It is the only continental landmass that covers the entire latitudinal range of the Southern westerly winds (SWW), whose interaction with the Southern Ocean play a key role in global climate. Seasonal temperatures and precipitation in Patagonia are strongly correlated with low-level zonal winds indicating that regional climate is mostly driven by the migrating locus of this hemispheric wind belt. Additionally, the Austral Andes generates a strong orographic effect that promotes a sharp transition between a western hyper-humid to an eastern semi-arid flank (Garreaud et al. 2013). Regional climate permits the existence of numerous mountain glaciers, including the Patagonian icefields, which constitute the most extensive ice masses of the Southern Hemisphere, outside Antarctica.
During the last glaciation, western Patagonia was covered by an ice sheet, which featured numerous outlet glacier lobes flowing towards both flanks of the Andes (Davies et al. 2020). These large glaciers formed a wide variety of ice-marginal landforms, including outstanding sets of moraines. This glacially shaped landscape has enabled Patagonia to play a major role in moraine-based paleoclimate reconstructions in the Southern Hemisphere.
Moraine-based T1 paleoclimate reconstructions in Patagonia
The end of the last glaciation, known as Termination 1 (T1), comprises a sequence of millennial-scale climate shifts that ultimately led global climate from cold-glacial to warm-interglacial conditions, between ~18 and ~11.7 kyr. δ18O from polar ice cores (Fig. 2b) exhibit a stepwise net warming trend interrupted by cold snaps between ~14.5–12.8 kyr in Antarctica, the so-called Antarctic Cold Reversal (ACR), and between ~12.7–11.7 kyr in Greenland, known as the Younger Dryas (YD). Millennial-scale climate shifts during T1 have been inferred as occurring synchronously, but in an out-of-phase style between hemispheres, which led to a proposed “thermal bipolar seesaw” as the potential mechanism responsible for this climate mode (Pedro et al. 2018). Therefore, deciphering the timing and geographical extent of both the ACR and the YD at planetary scale is imperative to properly test the influence of this hypothetical mechanism on global climate. To solve this fundamental paleoclimate conundrum, surface exposure chronologies of mountain glaciers offer crucial insights, including those from Patagonia.
Following pioneering cosmogenic dating of T1 moraines in Patagonia, recent highly resolved 10Be glacial chronologies are yielding unequivocal evidence of glacier pulses during the ACR and the YD from north to south. At Cerro Riñón valley in Lago Palena/General Vintter (44°S), Soteres et al. (2022) found moraine crests constructed between ~13.5–13.1 kyr and at ~12.4 kyr. In central Patagonia, Sagredo et al. (2018) documented moraine ridges formed between ~13.7–13.4 kyr and at ~12.0 kyr in the Río Tranquilo valleys around Cerro Cochrane/San Lorenzo (47.5°S). Furthermore, recent modeling analysis conducted by Muir et al. (2023) indicate that glaciers in Cerro Riñón and Río Tranquilo fluctuated in unison to a ~3°C cooling coeval with the ACR, followed by a ~0.5°C temperature increase coinciding with the YD. They concluded that glaciers in the northern half of Patagonia responded simultaneously to deglacial climate forcings. Nearby, in Lago Belgrano (47.8°S), Mendelová et al. (2020) reported moraines built between ~13.2–13.0 kyr and at ~12.4 kyr. In southern Patagonia, Ackert et al. (2008) and Kaplan et al. (2011) analyzed 10Be concentrations in moraine boulders while Strelin et al. (2011) used radiocarbon to date moraines at Lago Argentino (50°S), both constraining glacier advances culminating between ~13.2–13.0 kyr and at ~12.2 kyr. In the latter location, a Patagonian production rate for 10Be on rocks was also established, with implications for the accuracy of all Patagonian 10Be surface exposure ages. Further south, García et al. (2012) and Menounos et al. (2013) discussed moraines with ACR cosmogenic ages ranging between ~14.1–13.8 kyr in Torres del Paine (51°S) and ~13.4 kyr in Ushuaia (54°S), respectively (Fig. 2c).
Altogether, Bayesian 10Be ages probability correction of the Patagonian moraines reveal ubiquitous glacial advances/standstills in close morphostratigraphic order at least at ~14.0, ~13.4 kyr and ~13.0 kyr during the ACR, followed by pervasive ice retreat interrupted by a minor glacier pulse at ~12.2 kyr during YD times, spanning ~10° of austral latitude (Fig. 2d). Overall, these moraine chronologies show an emerging scheme that mimics millennial-scale climate shifts detected in both Antarctic and Greenland ice cores during T1 (Fig. 2b). This is supported by similar mountain glacier records obtained in the Northern Hemisphere (e.g. Bromley et al. 2023), challenging the widely accepted interhemispheric “thermal bipolar seesaw” mechanism. Denton et al. (2022) offered an alternative hypothesis that reconciles the apparent global synchronicity in mountain glacier fluctuations by invoking extreme seasonality episodes in the North Atlantic. Accordingly, the isotope signature in Greenland ice cores might be reflecting regional hyper-cold winters rather than a hemispheric climate signal, particularly during the YD. Their hypothesis, dubbed Heinrich Summers, implies that the Antarctic-like fashion of millennial-scale climate oscillations would have prevailed in both hemispheres during T1. If correct, this would favor the SWW-Southern Ocean coupled system as the main driver to generate and globally propagate climate signatures at millennial timescales during the end of the last glaciation.
Since early naturalists began to investigate glaciations in South America over a century ago, Patagonia has united several generations of scientists from all around the planet to establish the region as a key site for past glacier and paleoclimate studies. However, further research is needed to better understand the present-to-future evolution of global cryosphere and climate.
affiliationS
1Centro de investigación GAIA-Antártica, Universidad de Magallanes, Punta Arenas, Chile
2Centro Internacional Cabo de Hornos, Universidad de Magallanes, Puerto Williams, Chile
3Instituto de Geografía, Pontificia Universidad Católica de Chile (UC), Santiago, Chile
4Estación de Investigaciones Multidisciplinares Patagonia UC, Bahía Exploradores, Chile
5Lamont-Doherty Earth Observatory, Columbia University, Palisades, USA
6CICTERRA, CONICET – Universidad Nacional de Córdoba, Argentina
7Department of Earth and Environmental Sciences, Columbia University, New York, USA
contact
Rodrigo L. Soteres: rodrigo.soteresumag.cl
references
Ackert RP et al. (2008) Science 321: 5887
Bromley G et al. (2023) J Geophys Res 128: e2022JF006951
Davies BJ et al. (2020) Earth Sci Rev 204: 103152
Denton GH et al. (2022) Quat Sci Rev 295: 107750
García JL et al. (2012) Geology 40: 859-862
Garreaud R et al. (2013) J Clim 26(1): 215-230
Kaplan MR et al. (2011) Earth Planet Sci Lett 309: 21-32
Mendelová M et al. (2020) Quat Sci Rev 227: 106047
Menounos B et al. (2013) Quat Sci Rev 77: 70-79
Muir R et al. (2023) Quat Sci Rev 305: 108035
Pedro JB et al. (2018) Quat Sci Rev 192 : 27-46
Sagredo EA et al. (2018) Quat Sci Rev 188: 160-166
Schaefer JM et al. (2022) Nat Rev Methods Primers 2:18